Accelerator, Laser, and Coherent Optoelectronics R&D Lab (ALCOL)
Crystal Channeling Accelerator
Research on TeV/m CNT-Channeling Acceleration (ASTA)
The shock waves in an ionized plasma media, excited by relativistic particles, have been of great interest on account of their promise to offer extremely high acceleration gradients of G0 (max. gradient) = mecwp/e » 96 ´ n01/2 [V/m], where wp = (4pnpe2/me)1/2 is the electron plasma frequency and np is the ambient plasma density of [cm-3], me and e are the electron mass and charge, respectively, and c is the speed of light in vacuum. However, practically obtainable electron density (np) of plasmas in ionized gas is limited below ~ 1018 cm-3, which in principle corresponds to wakefields up to ~ 100 GeV/m, and it is realistically very difficult to create a stable gas plasma with a charge density beyond the limit. Metallic crystals are the naturally existing dense plasma media completely full with a large amount of conduction electrons available for the plasma wakefield interactions. The density of charge carriers (conduction electrons) in solids n0 = ~ 1020 – 1023 cm-3 is significantly higher than what was considered above in gaseous plasma, and correspondingly wakefield strength of conduction electrons in solids, if excited, can possibly reach 100 GV/cm or 10 TV/m in principle. Atomic channels in crystals are known to consist of 10 – 100 V/Å potential barriers capable of guiding and collimating a high energy beam and continuously focused acceleration with exceptionally high gradients (TeV/m). However, channels in natural crystals are only angstrom-size and physically vulnerable to high energy interactions. Carbon-based nano-crystals such as carbon-nanotubes (CNTs) and graphenes have a large degree of dimensional flexibility and thermo-mechanical strength, which could be suitable for channeling acceleration of MW beams. Nano-channels of the synthetic crystals can accept a few orders of magnitude larger phase-space volume of channeled particles with much higher thermal tolerance than natural crystals.
We investigated physical characteristics and beam dynamics of the channeling acceleration in hollow crystalline media like CNTs with theoretical analysis and effective plasma simulation based on particle-in-cell computation (e.g. VORPAL). The idea was explored regarding driving sources and material properties with various theoretical and numerical analyses. The concepts of laser-driven and beam-driven channeling accelerations were respectively published to the Physics of Plasmas and Applied Physics Letters. Based on the simulation results, we studied experimental feasibility of beam-driven acceleration by analyzing required beam parameters, including beam emittances, bunch charge, de-phasing length, etc, and structural tolerance of CNTs against the high power accelerations. Also, we conceived the idea of making the straight CNT up to 100 microns using CVD process on an anodic aluminum oxide (AAO) membrane template and designed CNT materials for the acceleration test, which is dimensioned with 100 nm in diameter and 100 mm in length. AAO-CNTs have been successfully fabricated in the collaboration with Nanolab Inc. We also designed the ASTA injector beamline for the feasibility test based on simulation-modeling with VORPAL, CST, and Elegant. The analysis was based on the ASTA nominal beam parameters (50 MeV and 3.2 nC), which indicated that a beam-driven self-acceleration produces 1 – 2 % net energy gain in the quasi-linear regime (off-resonance beam-plasma coupling, np = 1000 nb) with a 100 mm long effective CNT model. The outlined experimental plan with the simulation results was presented at the 16th Advanced Accelerator Concept Workshop (AACW2014) and the 6th International Conference - Channeling 2014 Charged & Neutral Particles Channeling Phenomena (Invited). Also, it was published to the Nuclear Instruments and Methods in Physics Research Section B: Beam Interactions with Materials and Atoms and AIP Proceedings. We joined the international collaboration for high field crystal accelerator R&D called “Transformative High Energy X-ray Acceleration in Crystal (THEXAC)”, including Fermilab, SLAC, UCI, IZEST, ELI, Ecole Polytechnique. Currently we are planning to test CNT-channeling at the Fermilab-ASTA injector beamline to be commissioned within a month.
Abstract
Atomic channels in crystals are known to consist of 10 – 100 V/Å potential barriers capable of guiding and collimating a high energy beam and continuously focused acceleration with exceptionally high gradients (TeV/m). However, channels in natural crystals are only angstrom-size and physically vulnerable to high energy interactions. Carbon-based nano-crystals such as carbon-nanotubes (CNTs) and graphenes have a large degree of dimensional flexibility and thermo-mechanical strength, which could be suitable for channeling acceleration of MW beams. Nano-channels of the synthetic crystals can accept a few orders of magnitude larger phase-space volume of channeled particles with much higher thermal tolerance than natural crystals. Our particle-in-cell simulations with 100 mm long effective CNT model indicated that a beam-driven self-acceleration produces 1 – 2 % net energy gain in the quasi-linear regime (off-resonance beam-plasma coupling, np = 1000 nb) with ASTA 50 MeV injector beam parameters. This paper presents current status of CNT-channeling acceleration experiment planned at the Advanced Superconducting Test Accelerator (ASTA) in Fermilab.
Introduction
Shock waves in an ionized plasma media, excited by relativistic particles, have been of great interest on account of their promise to offer extremely high acceleration gradients of G (max. gradient) = mecwp/e » 96 ´ n01/2 [V/m], where wp = (4pnpe2/me)1/2 is the electron plasma frequency and np is the ambient plasma density of [cm-3], me and e are the electron mass and charge, respectively, and c is the speed of light in vacuum. However, a practically obtainable plasma density (np) in ionized gas is limited to below ~ 1024 m-3, which in principle corresponds to wakefields up to ~ 100 GV/m [1, 2], and it is realistically very difficult to create a stable gas plasma with a charge density beyond this limit. Metallic crystals are the naturally existing dense plasma media completely full with a large number of conduction electrons available for the wakefield interactions. The density of charge carriers (conduction electrons) in solids n0 = ~ 1026 – 1029 m-3 is significantly higher than what was considered above in gaseous plasma, and correspondingly the wakefield strength of conduction electrons in solids, if excited, can possibly reach 10 TV/m in principle.
The channels between atomic lattices or lattice planes aligned in a crystal orientation of natural crystals like silicon or germanium are sparse spaces with relatively low electron densities. Charged particles, injected into a crystal orientation of a mono-crystalline (homogeneous and isotropic) target material, undergo much lower nucleus and electron scatterings. The idea of accelerating charged particles in solids along major crystallographic directions was suggested by several scientists such as Pisen Chen, Robert Noble, Richard Carrigan, and Toshiki Tajima in the 1980’s and 1990’s [3 – 6] for the possible advantage that periodically aligned electrostatic potentials in crystal lattices are capable of providing a channeling effect [7 – 9] in combination with low emittance determined by an Ångström-scale aperture of the atomic “tubes”. The basic concepts of atomic accelerator with short pulse driving sources like high power lasers or ultra-short bunches have been considered theoretically. However, the idea has never been demonstrated by experiment or simulation due to the extremely tight interaction condition of the Angstrom-size atomic channels in natural crystals and the complexity of electron dynamics in solid-plasma.
nanotubes for channeling
Carbon nanotubes (CNTs) are a synthetic nanostructure, which is a roll of a graphene sheet, and its tube diameter can be easily increased up to sub-micron by optimizing fabrication processes (chemical vapor deposition, CVD). For channeling applications of high power beams, carbon nanostructures have various advantages over crystals [10]. Particles are normally de-channeled when the transverse forces are larger than the maximal electric field acting on channeled particles from crystal atoms, which is described by the critical angle. The dechanneling rate is significantly reduced and the beam acceptance is increased by the large size of the channels, e.g., a 100 nm wide CNT channel has larger acceptance than a silicon channel by three orders of magnitude. If the channel size is increased from angstroms to nanometers, the maximally reachable acceleration gradient would be lowered from ~ 100 TeV/m to ~ 1 TeV/m due to the decrease of effective plasma charge density. However, the nanotube channels still provide sufficiently large transverse and longitudinal fields in the range of TV/m. For the crystal channels in angstrom scale, the lattice dissociation time of atomic structures (, where mi and me are the masses of ion and electron respectively [11]) is in the range of sub-100 fs with 1 TV/m fields, corresponding to 1019 W/cm3. For beam driven acceleration, a bunch length with a sufficient charge density would need to be in the range of the plasma wavelength to properly excite plasma wakefields, and channeled particle acceleration with the wakefields must occur before the ions in the lattices move beyond the restoring threshold and the atomic structure is fully destroyed. It is extremely difficult to compress a particle bunch within a time scale of femto-seconds since the bunch charge required for plasma wave excitation and the beam power corresponding to the time scale will exceed the damage threshold of the crystal. The disassociation time is, however, noticeably extended, to the order of pico-seconds, by increasing the channel size to nanometers because the effective plasma density and corresponding plasma frequency are decreased by a few orders of magnitude. The constraint on the required bunch length is thus significantly mitigated and the level of power required for an external driving source could be lowered by a few orders of magnitude, although the acceleration gradient will be lowered accordingly. Furthermore, dephasing length [12] is appreciably increased with the larger channel, which enables channeled particles to gain a sufficient amount of energy. The atomic channels in natural crystals, even if they provide extremely high potential gradients, are limited to angstroms and are unchangeable due to the fixed lattice constants. The fixed atomic spaces make the channeling acceleration parameters impractically demanding, but CNTs could relax the constraints to more realistic regimes. It is known that thermal conductivity of CNTs is about 20 times higher than that of natural crystals (e.g. silicon) and the melting point of a freestanding single-tip CNT is 3,000 – 4,000 Kelvin. Carbon based channels thus have significantly improved physical tolerance against intensive thermal and mechanical impacts from high power beams.
beam parameters for self-acceleration in a cnt-channel
As CNTs normally have 6 - 7 ´ 10-4 e/atom of electron density (re), effective charge density of a single-wall CNT (zigzag: metal) is nSWNT (= np) = (2mW)re/ucnt, where mW is the number of unit-cells in a unit column and ucnt is the volume of unit column. For multi-wall CNTs (MWNTs), the effective charge density (nMWNT) is given by, where n is the number of walls separated by the wall-to-wall distance (dw ~ 0.34 nm). Here, a radius of the ith CNT wall is defined as Ri = R - i×dw, where R is the radius of outmost CNT wall. The effective charge density of large SWNTs or MWNTs with R = 50 - 100 nm ranges np = 1023 – 1024 e/m3. A bunch passing through an accelerating medium undergoes self-acceleration in that a bunch-head quickly perturbs the charge distribution, giving rise to an oscillation, and a bunch tail gains an energy from the wakefield. The longitudinal field of the beam self-acceleration [13] with a Gaussian bunch is described by eW|| = (16pe2nb/k2b)[I0(kr)K2(ka) + (1/2)(1 – r2/a2) - (2/k2a2)]´ [cos(kz)-((z+b)/b)+(1/kb)sin(kz)], where nb is the bunch charge density, a is the bunch radius and b is the bunch length, and k is the plasma wave constant (k = wp/ub, where ub is the beam velocity). Figure 1 shows the longitudinal wakefield and energy gain graphs of a self-accelerated beam with the CNT electron density (np ~ 5 ´ 1023 e/m3) and bunch charge Qb = 3.2 nC. The ASTA beam of injector beamline has 0.1 – 1 mm of focused rms beam size and ~ 1 – 2.4 mm and 0.3 mm of bunch lengths without and with a bunch compression, respectively. Therefore, with these beam parameters it is expected to have up to 1 GeV/m of acceleration gradient and a few hundred keV of energy gain through a 100 mm long CNT channel, which corresponds to ~ 0.1 – 1 % of 20 – 50 MeV.
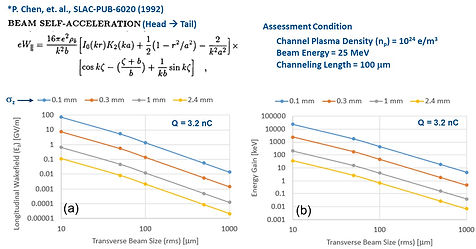
Figure 1: (a) Longitudinal wakefield (Ez = W||) and (b) energy gain versus transverse beam size of self-accelerated beam in a 100 long CNT channel with np ~ 5 ´ 1023 e/m3 (with different bunch lengths).
SIMULATION ANALYSIS WITH FERMILAB-ASTA 50 MEV BEAM
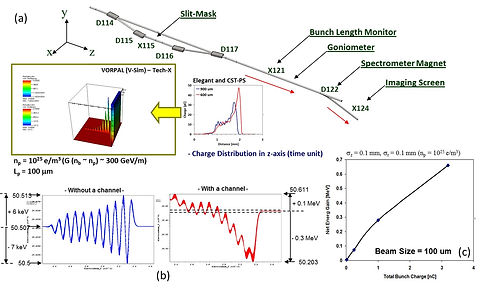
Figure 2: Simulation results with an effective CNT model along the ASTA injector beamline (a) beam-driven plasma wakefield simulation combined with the beamline model (Elegant and CST-PS) (b) longitudinal beam energy distributions (L) without and (R) with a CNT channel (L = 100 mm) (c) energy gain versus bunch charge with sr = 100 mm

The initial assessment of the prospective energy gains of CNT-channeled electrons was already fulfilled with a PIC-based beam-driven plasma simulator combined with the beamline simulations. For the simulations, the ASTA 50 MeV beamline from the chicane (BC1) to the imaging station (X124) was modeled with CST and Elegant (Fig. 2(a)). The two beam profiles of bunches with and without the modulation (modulation wavelength = lmb), which is generated by the slit-mask in the bunch compressor (BC1), are monitored at the goniometer position. The beam profiles are then manually imported to the effective CNT model. A typical simulation result (Fig. 2(b)) showed that a 1 nC bunch (uncorrelated energy spread = ~ 0.01 – 0.015 %, lmb = 100 mm) is self-accelerated with a net energy gain (~ 0.2 %) on the tail (witness) and an energy loss (~ 0.6 %) on the head (drive) along the 100 mm long channel with the nominal beam parameters. Our preliminary assessment with the full beamline model predicts that the ASTA 50 MeV beam can produce ~ 1 – 2 % of maximum net gain with 3.2 nC bunch charge and 100 mm transverse beam size (circular beam, Fig. 2 (c)), corresponding to 5 – 10 GV/m gradient. For the simulations, the bunch charge density is about a thousand times smaller than the channel charge density (off-resonance beam-plasma coupling). However, detecting the amount of energy gain by the proposed experiment will support feasibility of TeV/m acceleration in CNT channels. Generally, density modulations enhance energy efficiencies or power gains of coherent light sources or beam-driven accelerators. A beam, if longitudinally modulated, is more strongly coupled with accelerating or undulating structures at a resonance condition with the fundamental or higher order modes. Pre-bunched or modulated beams would improve the longitudinal beam control in energy-phase space and furthermore strongly enhance wakefield strengths or transformer ratio of beam-driven channeling accelerations [14]. In principle, a beam-density modulation (or micro-bunching) corresponding to an intrinsic channel plasma frequency possibly offers the optimum beam-plasma coupling condition with maximum energy transfer efficiency and acceleration gradient. The slit-mask modulation technique is relatively easy to generate a beam modulation.
Figure 3: From Paper
EXPERIMENTAL PERSPECTIVES
It is planned to test the CNT-channeling acceleration at the Fermilab ASTA 50 MeV beamline (Fig. 3). A 3 ~ 4 ps long electron bunch generated from the photo-injector is transported to the magnetic chicane (BC1). The bunch is compressed to ~ 1 ps by BC1, and the compressed beam will be focused by the quadrupole triplet magnets (Q118, 119, 120). After the beam spot size is focused to ~ 100 – 200 mm, it will be injected to a CNT target in the goniometer. The CNT targets will be fabricated by an anodic aluminum oxide (AAO)-CNT template process. [15]. The channeled electrons will be transported to an electron spectrometer consisting of a dipole (D122) and a screen in the energy dispersive region following D122at imaging station X124. Their energy distribution will be measured by the spectrometer before the beam is dumped to a shielded concrete-enclosure (beam dump). The pre-bunched beam generated by the slit-mask installed at X115 between two bending-dipoles (D115 and D116) will also be tested and the measured beam parameters will be compared with the ones of the bunched beam to check the impact of beam-modulation on channeling acceleration. Before testing the AAO-CNT target, the beam parameters will be characterized first without a target, which will be a reference for the beam-energy measurement. An experiment will be set up to check if the measured variation of the projected image on the screen due to presence of the target exceeds the nominal deviation of the image produced by the intrinsic energy spread. The initial experiment will then be followed by subsequent measurements to accurately identify net energy gains/losses and beam emittances: the channeled beam deflected by the magnetic spectrometer (D122) will be projected on the screen of the imaging station (X124). The beam-injection angle with respect to the target axis will then be scanned. A relative change of projected images from one angle to another will be translated into an energy gain/loss of channeled beam.
conclusion
Particle channeling in crystalline media has been considered an alternative promising technology for challenging scientific experiments at the high energy physics. Its strong atomic interactions along the crystal lattice planes enable efficient collimation/bending of intense beams and continuously focused acceleration with exceptionally large gradients up to a few tens of TeV/m. Development of the channeling acceleration concept has a great potential to advance accelerator technology for future HEP colliders.
acknowledgment
We thank P. R. G. Piot and Vladimir D. Shiltsev of Accelerator Physics Center (APC) in Fermi National Accelerator Laboratory (FNAL) and T. Tajima of University of California, Irvine for the helpful discussion on the idea and the support for the experimental plan.

Crystal Channeling: TeV/m Acceleration
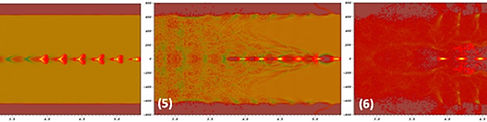
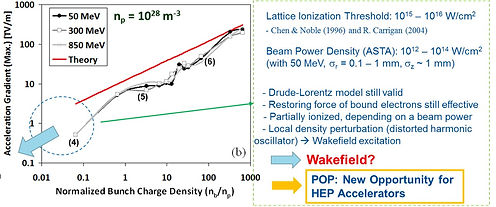
Beam-Driven Acceleration in Solid-Level Density
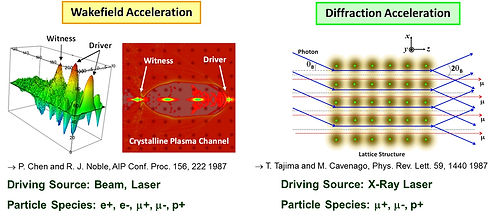
CNT's for Channeling Acceleration
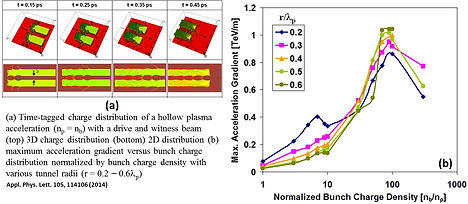
Beam-Driven Acceleration in effective CNT channel

CNTs for Channeling Acceleration

AA 0-CNTs: Sub-MM long Multi-Wall CNT's for channeling
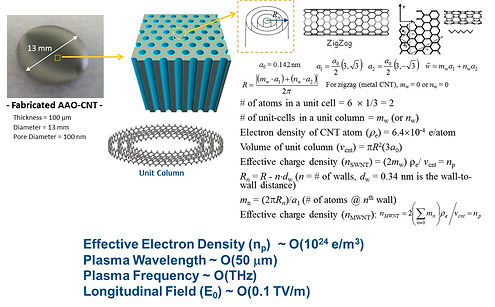
Effective Plasma (Electron) density of designed AA0-CN
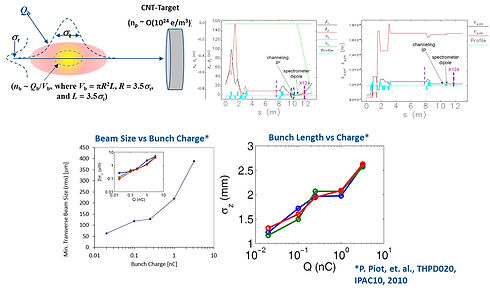
Estimate of Bunch Parameters
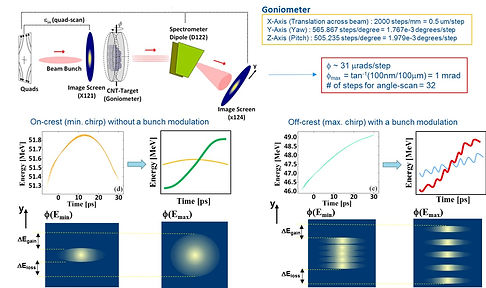
Identification of Wakefield Excitation and Energy Gain
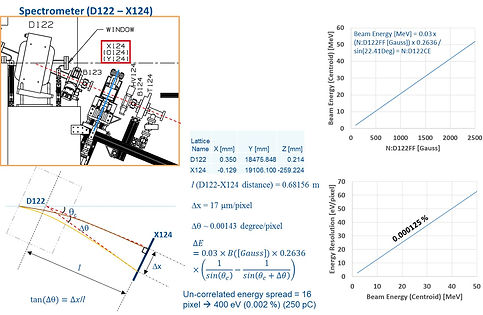
Energy Resolution of Spectrometer (D1222-X124)-Minumum Detectable Energy Variation